Class AB Amplifiers
- Boris Poupet
- bpoupet@hotmail.fr
- 10 min
- 27.701 Views
- 0 Comments
Introduction
Class A amplifiers offer a very good linearity of the output, meaning that the signal is faithfully reproduced, however their efficiency is very low, around 20-30 % in most of the cases. On the other hand, class B amplifiers present a very high efficiency up to 78.5 % but cannot reproduce the output faithfully. Indeed, a crossover distortion appears in a push-pull configuration and the use of a negative feedback is required to limit this effect.
In order to combine both the excellent linearity of class A and the high efficiency of class B, the class AB has been developed. In the very first section, we will present the general functioning and characteristics of class AB amplifiers. Thereafter, we will discuss the efficiency of class AB configurations. The following section will present some possible biasing methods and we will highlight that the diode biasing is the most appropriate. Finally we will see that even the diode biasing method needs to be improved in order for the class AB configuration to correctly amplify the signal with no crossover distortion.
Presentation of the Class AB amplification
As the name refers to, class AB is working half way between class A and class B amplifiers. The Figure 1 below shows the operating zone of class AB amplifiers in a (Vout,Iout) characteristic graph.
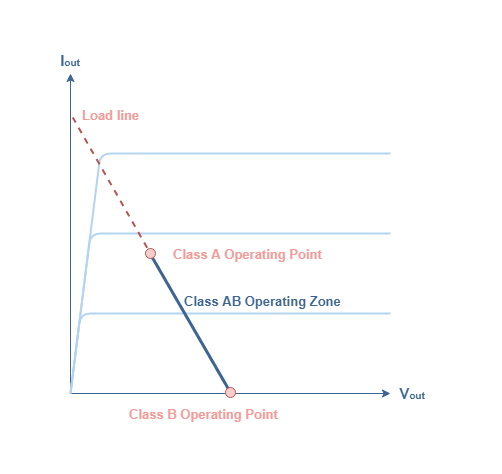
Class AB amplifiers can be biased by choosing an operating point along this blue line, excluding the class A and class B biasing points. The choice of its location depends on the desired levels of efficiency and linearity. If the class AB operating point is closer to the class A operating point (resp. class B) than the class B operating point (resp. class A) the circuit will behave more such as a class A amplifier (resp. class B) by presenting a higher linearity but a lower efficiency (resp. higher efficiency but lower linearity).
The conduction angle of class AB amplifiers is therefore in the ]180°,360°[ range. This type of amplifier conducts the signal more than 50 % of the time but less than 100 % such as shown in the Figure 2 below :
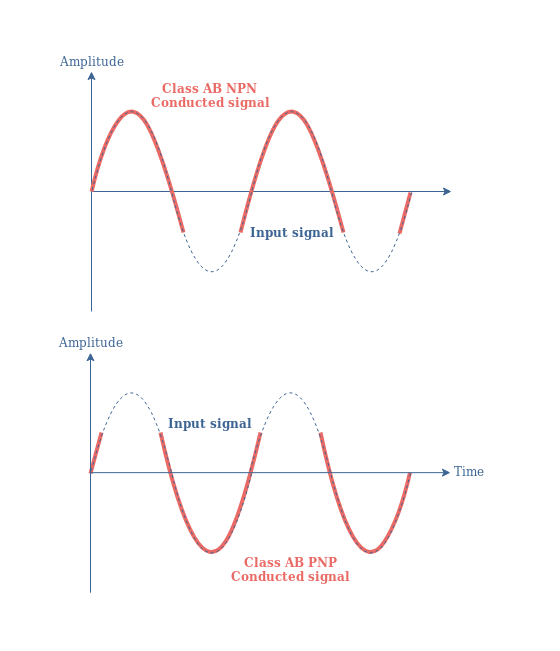
Both NPN and PNP-based class AB behaviors are presented since a push-pull configuration is necessary, such as for the class B amplifier, to combine the positive and negative half-waves in order to reproduce the complete signal.
We can note that a small portion of the signal is conducted simultaneously by the NPN and the PNP transistors. This ensures that no crossover distortion is observed during the amplification performed by class AB amplifiers.
Class AB efficiency
The formula of the efficiency (η) for class AB amplifiers is similar to the class B configuration and is given by the following equation :
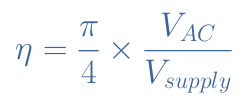
Where VAC represents the AC fluctuation of the output signal. The maximum efficiency here, not only depends on the maximized value of VAC, but also on the position of the operating point along the operating zone (see Figure 1) :
- If the class AB amplifier is biased at the limit of the cutoff point, VAC,max=Vsupply and thus ηmax=π/4=78.5 %.
- If the class AB amplifier is biased at the limit of the class A operating point, VAC,max=Vsupply/2 and thus ηmax=π/8=39.3 %.
- In the other cases, the maximum efficiency of a class AB amplifier will be in the ]39.3 % ; 78.5 %[ range.
Biasing methods
There are many ways of biasing a class AB amplifier in order to create an interval of voltage where the complementary transistors conduct simultaneously. In this section, we will briefly present some of the possible biasing methods by highlighting their advantages and disadvantages. The methods will be sorted by how relevant they are, we will indeed begin by the most inappropriate to end with the most common, easier to implement and efficient.
Voltage biasing
A very easy and intuitive method is to bias directly and independently the bases of the NPN and PNP transistors. This supply can be done by batteries or via a DC generator. The output stage of a push-pull configuration that includes this biasing method is shown in the Figure 3 below :
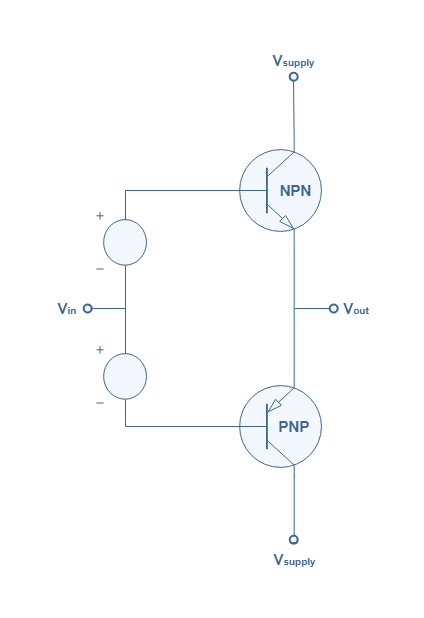
This method has the merit to work but is completely unadapted for real circuits. Indeed, due to packaging and cost constraints, no manufacturer is implementing this solution.
Voltage divider network
This method has been presented many times during the BJT amplifier tutorials and involves only passive components. The Figure 4 below presents a push-pull configuration associated with two voltage divider networks to bias the base of both transistors.
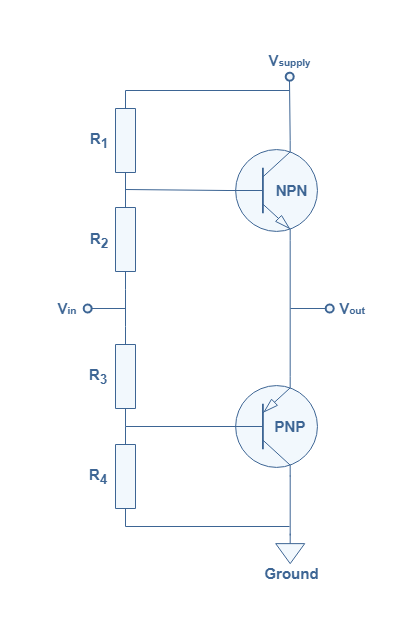
With this configuration, a voltage drop across the two bases (across the total resistance R2+R3) of 1.2 to 1.4 V is observed. This value corresponds to 2×VBE where VBE represents the threshold voltage of the transistors. This voltage drop will bring both transistors above the cutoff state, they will therefore both conduct simultaneously a portion of the input signal.
The main reason why this configuration cannot be used for class AB amplifiers is that the set of values of the resistors will only work for a particular push-pull configuration. Moreover, the set of values needs to be chosen very carefully, specially if the complementary transistors are different.
Potentiometer biasing
This method is very similar to the voltage divider network. The only difference is that an adjustable resistance (or potentiometer) is placed between the two networks. The advantage of this solution is to closely match the biasing of the two complementary transistors with a controllable resistance, even if the transistors have different electrical properties.
However, this solution as well as the voltage divider network involve resistor that generate heat dissipation by Joule’s effect. Since the class AB amplifiers are often biased slightly above the cutoff point to offer a maximum efficiency, any temperature change caused by this phenomenon can result in an inappropriate behavior of the push-pull configuration. This is the reason why diode biasing if often preferred as we will see in the next paragraph.
Diode Biasing
Above a certain value of current, diodes have the property to generate a constant voltage drop of ∼0.7 V. It is this feature that is exploited to provide a constant potential of 1.4 V between the two transistor’s bases as shown in the Figure 5 below :
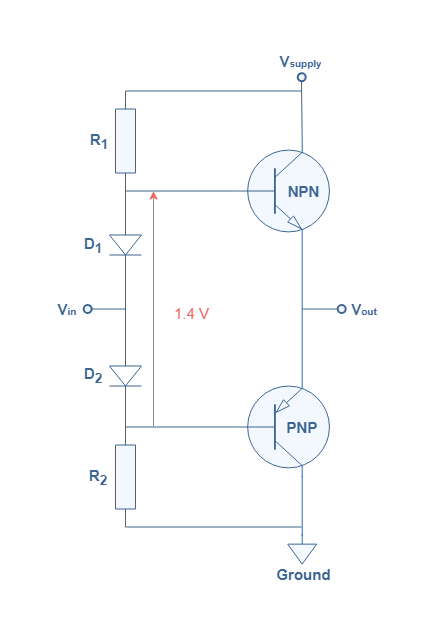
Another advantage of this configuration is that the voltage drop across the diodes is self-adjusted with any change of temperature. A rise of temperature will indeed have the effect to decrease the diodes threshold which will decrease the biasing of the transistors and therefore limit a possible thermal runaway.
Diode network and current source
The diode biasing circuit, as we just presented before, is the most adapted to bias class AB amplifiers. However biasing directly the diodes with resistances such as presented in Figure 5 leads also to some problems.
The main limitation comes from the resistances R1 and R2 that bias the diodes. Indeed, if a high output signal is needed, the bases of the transistor will collect all the current from the resistances, therefore the current in the diodes will be zero. Since the diodes receive no current, no bias will be delivered to the push-pull configuration and the crossover distortion will again be observed. This phenomenon is amplified by the fact that when the output voltage increases, the current across the resistances R1 and R2 decreases.
To solve this problem, the resistances are instead replaced with common emitter amplifiers that act as a current sources such as shown in Figure 6. In this case, the current sources can anticipate the variations of the output by providing a constant current as opposition to the resistances. Both the bases and the diodes can therefore be supplied with current.
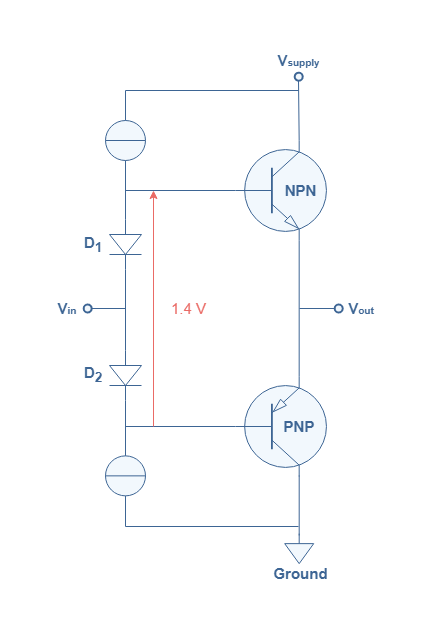
Power distribution for class AB amplifier
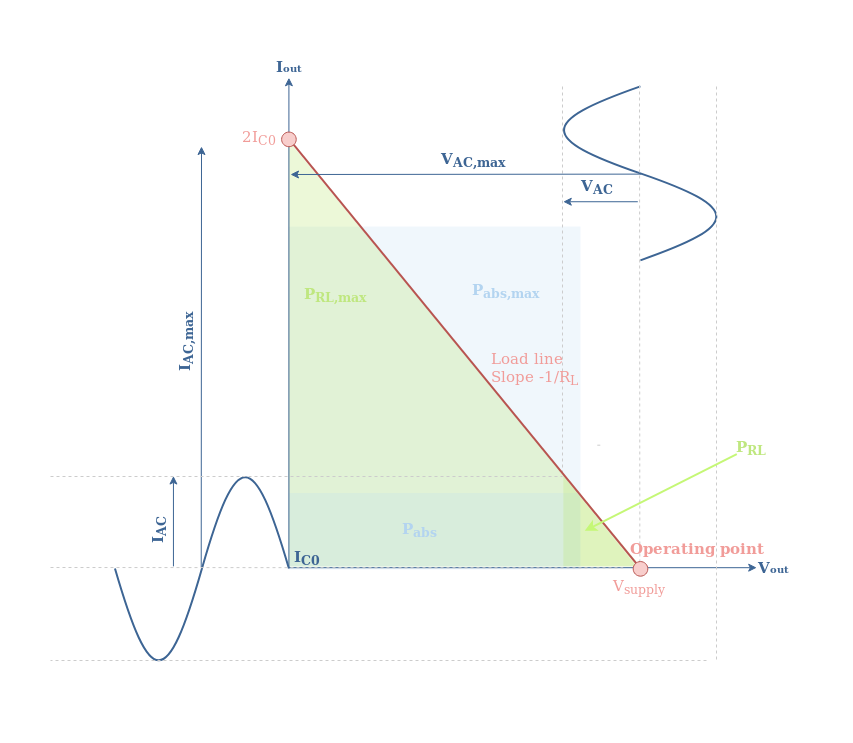
Conclusion
We have seen that class AB amplifiers contrast with class A and class B amplifiers since they do not have a unique operating point but rather an operating zone delimited by the class A quiescent point and the cutoff point (class B operating point). The location of the operating point along this zone will dictate both the conduction angle and the efficiency of the amplifier.
Indeed, if the class AB operating point is located closer to the class A operating point, the amplifier will have a conduction angle in the [270° ; 360°[ range, therefore conducting more than three quarters of the signal. Moreover, an efficiency in the ]39.3 % ; 58.9 %] interval will be observed.
In the other hand, if the operating point is located closer to the class B operating point, the amplifier will behave more likely as a class B by presenting a lower conduction angle in the interval ]180° ; 270°] which will increase its efficiency between 58.9 % and 78.5 %.
We have seen that a variety of different methods exist in order to bias the bases of a push-pull configuration. We have specifically focused on the voltage biasing, resistor-based biasing and diode biasing. The first one consists of directly biasing the bases with a voltage generator or batteries, but it is not implemented due to costs and packaging problems that arise with it. The second and third methods use voltage divider networks and modified networks with a potentiometer. However, these biasing circuits consist of many resistances and therefore cause heat dissipation that affects directly the behavior of the transistors.
The last method presented can overcome this problem by using a diode divider network. We have indeed seen that this structure creates a constant 1.4 V voltage drop between the two transistor’s bases, which is enough to create an interval where the NPN and PNP transistors conduct simultaneously. Moreover, diodes have the advantages over the resistor to cushion temperature fluctuations.
Finally, we have seen that the diode network must be biased with current sources in order to provide a constant bias to the push-pull configuration bases despite output variations.
The class AB configuration is nowadays the most common in electronic circuits since it combines the advantages of class A and class B amplifiers without their disadvantages.